The Quantum Biology Revolution


How Quantum Mechanics Could Unlock New Pathways for Cellular Repair and Aging
In recent years, the field of biology has experienced a paradigm shift with the introduction of quantum mechanics into its theoretical and experimental frameworks. Quantum biology, an emerging interdisciplinary field, seeks to understand how quantum phenomena might influence biological processes at the molecular and cellular levels. While the concept of quantum mechanics—typically reserved for the realms of physics and chemistry—may seem far removed from the biological world, recent discoveries suggest that these principles could play a crucial role in cellular repair, aging, and even the potential reversal of age-related decline.
Understanding Quantum Mechanics in Biology
Quantum mechanics is the branch of physics that deals with phenomena occurring at the atomic and subatomic levels. It describes how particles like electrons and photons behave, often in ways that defy our classical understanding of physics. For instance, particles can exist in multiple states simultaneously (a concept known as superposition) and can become entangled, meaning the state of one particle is instantaneously connected to the state of another, regardless of distance.
For decades, the idea that such principles could have any relevance to biology was met with skepticism. Biology, after all, deals with larger, more complex systems where classical physics reigns supreme. However, evidence is mounting that quantum phenomena may indeed have significant implications for biological processes, particularly those involving molecular interactions and energy transfers.
Quantum Coherence in Photosynthesis
One of the earliest and most compelling examples of quantum mechanics in biology comes from the study of photosynthesis. Researchers discovered that the energy transfer within the photosynthetic complexes of plants, algae, and certain bacteria exhibits quantum coherence. This means that the energy, in the form of excitons, can exist in a superposition state, allowing it to simultaneously explore multiple pathways to find the most efficient route to the reaction center where it is converted into chemical energy.
This discovery challenged the classical view of energy transfer as a random, diffusive process and suggested that quantum effects could make biological processes more efficient. If nature can harness quantum mechanics to optimize photosynthesis, what other biological processes might be influenced by these principles?
Quantum Tunneling and Enzyme Activity
Another area where quantum mechanics appears to play a role is in enzyme activity. Enzymes are proteins that act as biological catalysts, speeding up chemical reactions essential for life. One of the ways they achieve this is through a process known as quantum tunneling.
Quantum tunneling allows particles to pass through energy barriers that, according to classical physics, should be insurmountable. In the context of enzyme activity, this means that protons or electrons can “tunnel” through the energy barrier of a reaction, significantly increasing the reaction rate. This phenomenon is particularly important in processes like cellular respiration and DNA replication, where enzymes must operate with incredible speed and precision.
Implications for Cellular Repair and Aging
The potential applications of quantum biology extend far beyond understanding basic biological processes. One of the most exciting possibilities lies in the realm of cellular repair and aging. Aging is often associated with the accumulation of damage at the cellular level, particularly in DNA and other critical biomolecules. If quantum mechanics can influence processes like enzyme activity and energy transfer, it raises the question: Could these principles be harnessed to improve cellular repair mechanisms and slow down aging?
Quantum Effects in DNA Mutations and Repair
DNA, the blueprint of life, is constantly subjected to damage from environmental factors like UV radiation, toxins, and even normal metabolic processes. Cells have evolved sophisticated repair mechanisms to fix this damage, but these processes are not perfect, and errors can accumulate over time, leading to mutations and aging.
Recent research suggests that quantum mechanics might play a role in both the occurrence and repair of DNA mutations. For example, quantum tunneling could contribute to spontaneous mutations by allowing protons in the DNA bases to shift positions, creating tautomeric forms that pair incorrectly during replication. On the other hand, understanding these quantum effects could also lead to new strategies for enhancing DNA repair mechanisms, potentially reducing the rate of aging-related mutations.
Quantum Entanglement and Cellular Communication
Another intriguing possibility is the role of quantum entanglement in cellular communication. Cells constantly communicate with each other through a complex network of signaling pathways. While this communication is typically understood in terms of biochemical signals, some researchers speculate that quantum entanglement could allow for even more efficient and instantaneous information transfer.
If true, this could revolutionize our understanding of how cells coordinate responses to damage and stress. Enhancing this communication could improve the efficiency of cellular repair processes, reducing the impact of aging and potentially extending healthy lifespan.
Challenges and Future Directions
While the potential of quantum biology is immense, it is important to recognize that the field is still in its infancy. Many of the proposed quantum effects in biological systems are not yet fully understood, and there is ongoing debate about their significance. Additionally, translating these theoretical concepts into practical applications for medicine and aging presents significant challenges.
One of the primary challenges is the complexity of biological systems. Unlike the relatively simple systems studied in quantum physics, biological organisms are highly complex, with countless interacting components. Isolating and studying quantum effects in such systems requires sophisticated experimental techniques and interdisciplinary collaboration between physicists, biologists, chemists, and engineers.
Another challenge is the delicate nature of quantum states. Quantum phenomena are notoriously difficult to observe and control because they are easily disrupted by their environment. Biological systems operate at temperatures and conditions that are far from the cold, isolated environments typically required for observing quantum effects. Developing methods to harness quantum mechanics in these conditions will be a significant hurdle.
Despite these challenges, the potential rewards are too great to ignore. As our understanding of quantum biology deepens, it could lead to breakthroughs in fields ranging from medicine to biotechnology. For example, new quantum-based therapies could be developed to target specific cellular processes involved in aging and disease, offering more precise and effective treatments.
Conclusion: A New Frontier in Biology
The intersection of quantum mechanics and biology represents a new frontier in science, one that challenges our traditional understanding of life at the molecular level. While much work remains to be done, the early findings in quantum biology suggest that quantum mechanics could play a pivotal role in processes like cellular repair and aging.
If these principles can be harnessed, they could open up new pathways for extending healthy lifespan, repairing damaged tissues, and even reversing some aspects of aging. As researchers continue to explore this fascinating field, the future of medicine and aging could be profoundly shaped by the strange and wondrous world of quantum mechanics.
Share Your Success Story
Be Our Inspiration
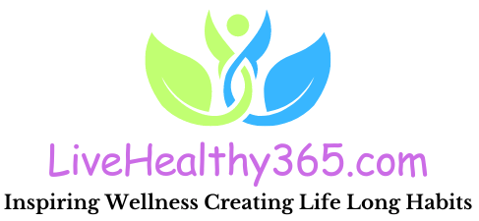
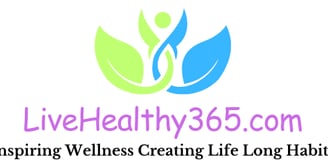